

The values of isotropic and anisotropic chemical shift parameters accumulated so far have been utilized to reveal either secondary or 3D structures of fibrous, membrane and globular proteins in solid-state or aqueous solution-state. These CSA parameters for nuclei in challenging proteins are now also available from samples using fast magic angle spinning (MAS) experiments. Chemical shift anisotropies (CSAs) of individual nuclei were initially obtained by solid-state NMR studies on single crystalline, static, or slowly rotating samples. Detailed analyses of such data showed that these chemical shift parameters are indispensable restraints for determining 3D structures of large proteins with or without the usual NOE constraints. The existence of conformation-dependent chemical shifts was initially recognized for a variety of biological molecules by solid-state NMR studies on polypeptides, polysaccharides, antibiotics, and so on and later by a careful examination of a robust database of chemical shifts available from multidimensional NMR studies of globular proteins in aqueous solution.

It is therefore very important to gain insights into how and to what extent the isotropic or anisotropic chemical shift of a nucleus under consideration are related to molecular and electronic structure. It is emphasized here that we are now in an era when solid-state and solution NMR approaches are frequently utilized in the analyses of CSA parameters and their applications for structural and dynamical studies of biomolecules. Such data are currently available from the literature. Determination of residue-specific 15N CSA for proteins in solution is now feasible by careful evaluation of T 1 and T 2 values at various external magnetic fields. This has stimulated much interest in evaluating the CSA values of nuclei in individual amino acid residues of large globular proteins in aqueous solutions or micelles. For this purpose, it is essential to have a prior knowledge of the magnitude of the CSA values for nuclei under consideration to optimize the condition for TROSY-type experiments. Under such circumstances, transverse relaxation-optimized spectroscopy (TROSY) can be utilized to record well-resolved spectra of large proteins or protein complexes with molecular weight on the order of 100 kDa, with the advantage of an attenuated T 2 relaxation by mutual cancellation of dipole-dipole coupling and CSA under the proton-coupled condition. The contribution of the CSA to T 2, and hence the width of the resonance increases with magnetic field strength leading to broad, unresolved lines.
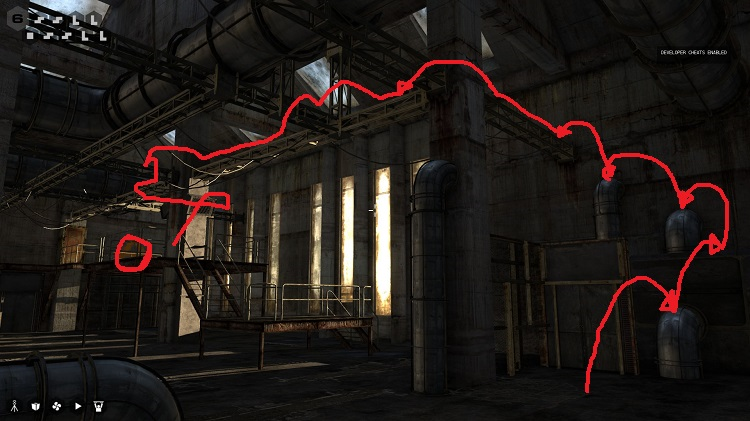
It should be mentioned that CSA is an important source of spin-lattice (T 1) and spin-spin (T 2) relaxation at high magnetic fields even for solution samples, although not affecting the shapes of solution NMR spectral lines. The chemical shift anisotropy (CSA) is usually obtained from NMR experiments on a solid or a liquid crystalline sample the corresponding isotropic chemical shifts can also be measured from solid samples. They are undoubtedly one of the most important NMR parameters measured from high-resolution NMR experiments, besides other parameters such as spin coupling constants and nuclear relaxation times, for determining primary or secondary structures of molecules, their dynamics, intermolecular interactions, etc. Isotropic chemical shifts of nuclei in different chemical groups of a molecule, are usually available from a solution sample where there is motional averaging of the anisotropic shielding tensor. In such cases, it should be bore in mind that Δδ = − Δσ and σ 33 ≥ σ 22 ≥ σ 11. Nevertheless, there are many previous papers utilizing shielding constants δ i’s, instead chemical shifts δ’s. Undoubtedly, the nomenclature based on the chemical shift parameter δ ii is directly related to the chemical shift anisotropy (CSA) which is one of the main topics discussed in this article.
